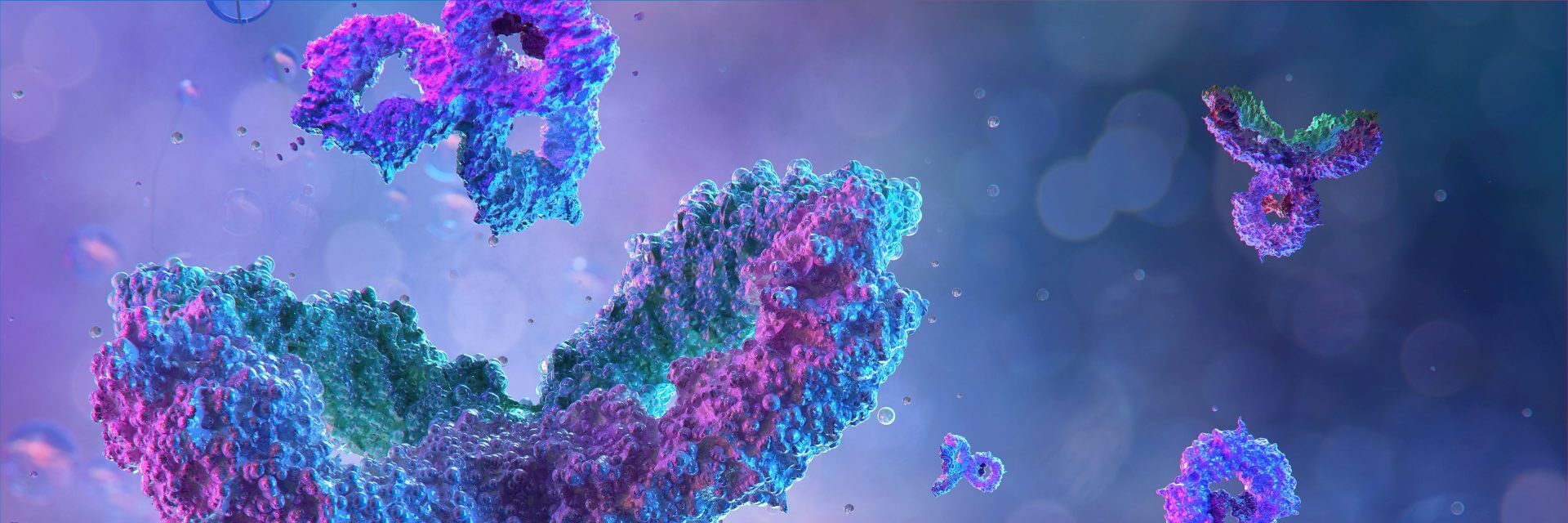
How do classic plasma fractionation separation methods compare? And what comes next?
World War II demonstrated the value of human serum albumin in battlefield casualties and cases of shock. Albumin was identified as a quick and efficient way to treat blood loss, but this required separating albumin from plasma. In the 1940s, at the request of the U.S. government, a method was developed by Professor Edwin Cohn of Harvard University which is still in use today. He established techniques for isolating the serum albumin fraction of blood plasma using cold ethanol. Over 70 years later, plasma fractionation methods are still largely unchanged.
Plasma fractionation is the downstream processing of plasma that has been harvested by donors. It breaks plasma into individual proteins, or plasma fractions. (It should be noted that this is different from whole blood fractionation, which means taking whole blood and breaking it into its four main parts.)
Over time, the scope of plasma fraction use has expanded and there are now over 20 different proteins that are of interest to the healthcare industry and therapeutics/biologics. Currently, large scale manufacturers are especially focused on:
Albumin
Albumin is still a mainstay of trauma medicine. These blood fractions make up the bulk of blood and maintain osmotic pressure in the body. During a trauma involving large fluid loss, albumin is often used as an immediate treatment.
Immunoglobulins (IgG)
These antibodies form the basis of the human immune system and are formed through the natural biological process of the body recovering from an attack of an infectious organism (eg. COVID-19). Immunoglobulins are also sometimes the sole treatment for rare conditions such as primary immunodeficiency.
Coagulation factors
These blood fractions are used in the treatment of blood clotting disorders, such hemophilia and von Willebrand’s disease.
Protease inhibitors
Emphysema can be caused by lung damage triggered by alpha-1 antitrypsin (AAT) deficiency. Purified human alpha-1-proteinase inhibitors are used as an effective treatment.
How does plasma fractionation work?
Most separation methods of blood plasma proteins are based on an initial altering of the physical and chemical properties of plasma or compounds within. This altering of properties leads to a change in solubility, and, ultimately, the precipitation of target plasma proteins within the liquid plasma solution. In some instances, the precipitation of plasma proteins is achieved through a controlled temperature change, known as cryoprecipitation. Most protein precipitation requires the addition of a precipitation or separation agent. Once the protein has precipitated, the protein can be isolated by mechanical or chemical separation methods.
The first well-established separation agent for large scale plasma processing was cold ethanol, and most of the plasma manufacturing industry still uses cold ethanol fractionation. However, depending on which protein is being targeted and whether crude plasma or plasma intermediates are being processed, other separation agents may be used, such as caprylic acid, ammonium sulphate, and polyethylene glycol—which all affect the purity and quality of specific proteins differently. In the case of crude plasma processing, a combination of cryoprecipitation, ethanol fractionation, and mechanical separation still forms the backbone of many plasma fractionation processes.
Mechanical separation methods in crude plasma processing
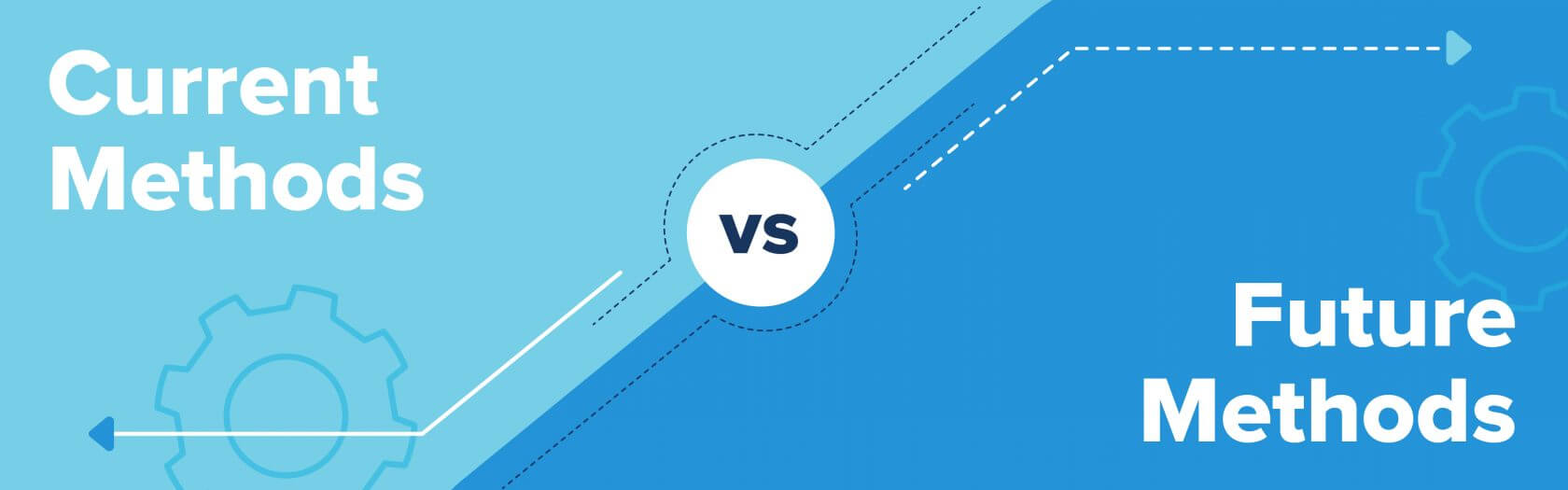
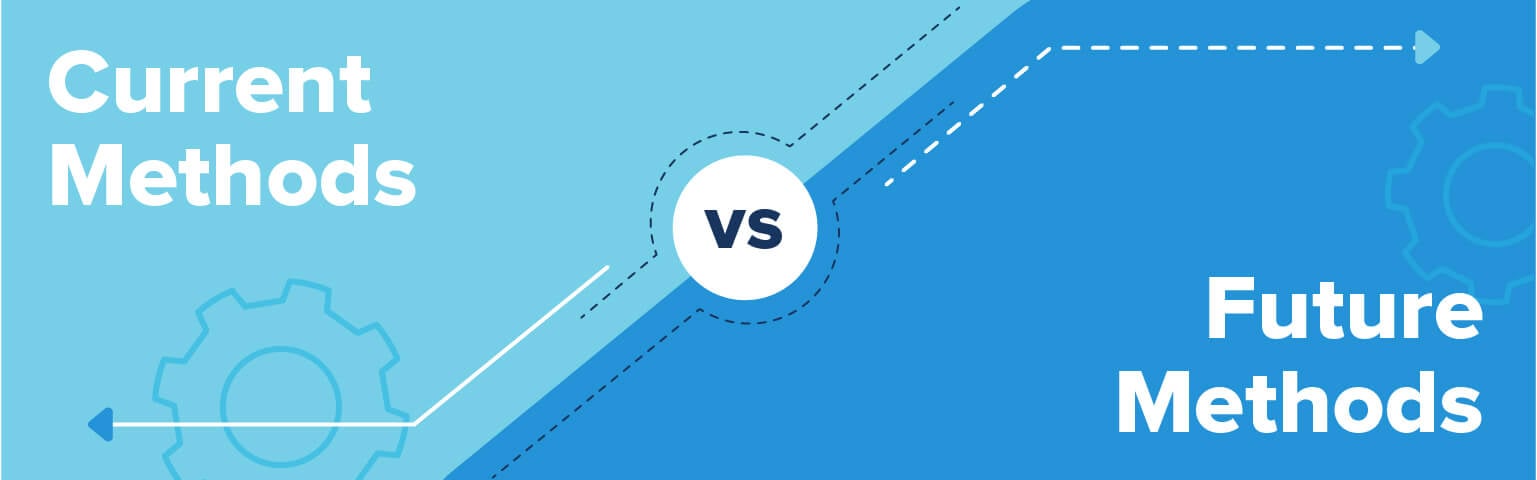
Centrifuges
Centrifuges were the main separation equipment used for decades and are still used extensively today. The G-forces created by their spinning motion separates based on differences in density. The proteins that have precipitated have a higher density than the solubilized liquid plasma and remaining soluble proteins. The solid protein precipitates are retained within the centrifuge while the liquid plasma and remaining soluble proteins are allowed to pass through the centrifuge. Subcategories of centrifuges include multi-chamber bowls (used in large scale fractionation), tubular centrifuges (used in small-scale operations, such as a lab setting), and disc stack centrifuges (common in biomanufacturing but not widely used in plasma fractionation).
Depth Filtration
Another method of plasma protein separation is depth filtration. Depth filters are made up of an internal structure that creates a tortuous path, causing precipitated plasma proteins to become trapped or adsorbed within the filter structure. As for depth filtration, filter presses form the backbone of this category when applied to large scale plasma protein separation. Other forms of depth filtration that have seen application in plasma purification processes include cartridge filter housings and rotating disc filtration.
Chromatography
Packed bed chromatography is a well-established technique that is widely used in the downstream purification of plasma protein intermediates that have already been separated from the crude plasma by the previously mentioned separation techniques. Packed bed chromatography traditionally has not been well suited for crude plasma purification primarily due to the shear volume of proteins present, resulting in a required chromatography resin volume that is cost prohibitive for most large-scale manufacturers.
If anything stands a chance to replace the classic ethanol precipitation and mechanical separation approach in the future, it will likely be through technological advancements in large scale chromatography techniques. One of the more promising solutions is the use of expanded bed adsorption chromatography. This is still not a cost-effective solution for most large-scale plasma fractionators. Until then, ethanol fractionation and mechanical separation is here to stay.
Which separation method is best?
The first separation step of plasma fractionation, known as the cryoprecipitate step, is nearly always performed in a multi-chamber centrifuge. After that step, however, the process becomes much more variable in terms of common practices used in modern fractionation facilities.
The discussion below considers the pros and cons of two of the most common methods: using a multi-chamber bowl centrifuge versus a standard plate and frame filter press. These recommendations are based on using cold ethanol as the anti-solvent agent to precipitate the sought-after proteins such as immunoglobulins, albumin, and coagulation factors. Depending on the protein of interest, a different agent and/or separation method may be more suitable.
Capital costs
One of the common drivers for deciding which separation method to use is equipment capital cost. From an initial cost point-of-view, filter presses and centrifuges have similar price tags on a per unit basis. However, in a typical large-scale operation, only one filter press is typically needed while two to three centrifuges are required to process the same plasma volume.
Next, consider the cost of supporting equipment. A simple manual filter press does not require much supporting equipment beyond the usual clean utilities systems found in most GMP facilities, such as Clean-in-Place (CIP) systems. A centrifuge, however, often requires multiple dedicated cooling systems, as well as dedicated cleaning equipment in addition to CIP. Ensuring satisfactory plasma protein yield and quality requires very accurate temperature control throughout the separation process. The temperature setpoint varies depending on the target protein, but in some instances it can be as low as -10°C. The resultant required temperatures for some of the cooling systems can be as low as -35°C, making the cooling equipment used to achieve these very cold temperatures complex and expensive.
The standard plate and frame filter presses designed by most manufacturers for the plasma industry are manually indexed and cleaned, while the piping is cleaned with an automated CIP cycle. Chamber centrifuges have a large solid bowl that must be manually disassembled for cleaning. Some manufacturers rely on time- and labor-intensive manual cleaning while others use automated cleaning equipment, such as a Clean-Out-of-Place (COP) cabinet to wash the centrifuge bowl. There are further costs associated with the carts used to transport the bowl back-and-forth to the cleaning chamber. Factor in the need for multiple washers and carts for multiple centrifuges, and the cost quickly rises.
It is also worth noting, however, that the facility’s philosophy for handling of filter aid for filter press operations is an important consideration that can lead to additional equipment costs for the filter press option. Some facilities have procedures for manual weighing and manual addition of filter aid, in which case capital costs for filter aid equipment is minimal. Others may choose to adopt dispensing and pneumatic conveying systems that automatically transport filter aid to the required use points, substantially increasing equipment capital costs.
Lower capital costs: Filter press
Facility footprint
When considering which method to employ in any facility, it is also important to look at how much space the equipment will occupy within the facility. When retrofitting an existing facility, available space can be very limited. For a greenfield facility, the required footprint to house all of the equipment can quickly drive up the overall facility and operating cost, especially considering the cost premium for any cleanroom space requirements.
The footprint of a single filter press is larger than that of a centrifuge. But that isn’t the whole story—one must look at the bigger picture of the support equipment required by centrifuges, as well as the possibility of needing multiple centrifuges. When considering the additional cooling and cleaning equipment required for centrifuges (summarized in the previous section), the required facility footprint for the centrifuge option is substantial. The cleaning and cooling systems often also need to be located in close proximity to the centrifuge(s) to maximize efficiency.
A filter press, however, doesn’t have any direct cooling. Instead, in order to meet cold temperature requirements, it must be installed in a refrigerated cleanroom. While a standard cleanroom already carries a cost premium, a refrigerated cold cleanroom will be even more expensive to build and operate due to the required insulated wall panel construction, as well as any additional refrigeration equipment and additional dehumidification requirements for the HVAC system that supports that room. A centrifuge may not require a cold room as it has direct cooling built in. However, there are some instances where a centrifuge will still need to go into a cold room, depending on the protein of interest and whether that protein is manually harvested at the centrifuge, or if it remains in the liquid supernatant that passes through the centrifuge.
All things considered, the facility footprint required for either a chamber centrifuge or a standard plate and frame filter press turns out to be about the same once space requirements for cooling and cleaning equipment, cold rooms, and clean rooms are factored in.
Facility footprint: Comparable
Complexity
Next, complexity needs to be considered. This pertains to the degree of interaction operators need to have with the technology used, as well as the overall complexity of the equipment design. Before deciding upon a manual filter press or a multi-chamber bowl centrifuge, ask: ‘How much manual operator intervention is acceptable?’ Facility owners can have varying opinions on how much manual intervention is appropriate for the operation of process equipment. Some prefer to keep the process as manual as possible to minimize capital costs for automation systems, while others prefer to avoid operator interaction all together to improve process repeatability, reduce labor costs, and improve operator safety. Automated processes are also typically subject to less scrutiny by regulatory agencies since they are viewed as more reliable and less subject to human error.
A manual filter press is the simpler of the technologies, while a centrifuge has a lot of parts and pieces that need to be manipulated on a daily basis. A centrifuge requires several manual operations. For example, its bowl needs to be manually disassembled, removed, and placed on a cart for cleaning after each use. This is no easy feat— a bowl weighs several thousand pounds and needs to be removed and then rolled through hallways by several operators into the COP washer. Once cleaning is complete, the bowl then needs to be wheeled back through the facility to the centrifuge room and reassembled piece-by-piece before operation can resume.
Additionally, the large G-forces generated by the centrifuge requires additional safety features within the equipment design, such as internal vibration safety sensors that trigger automatic shut down if the machine is vibrating excessively due to an imbalanced load or mechanical failures within the machine. This, among other design features of the centrifuge, means the centrifuge will generally require more maintenance than a filter press.
When it comes to protein harvesting, the filter press requires a similar degree of manual intervention. But the operating principle of a standard plate and frame filter press by contrast is much simpler than a centrifuge. Typically, a hydraulic unit is used to maintain some compression of the filter press plates to provide a liquid seal. After a batch has been processed, the hydraulic unit releases pressure and allows operators to manually index and clean the plates which are kept in place by a rail system. Typically, all cleaning and operation take place in one location and there are fewer moving parts and pieces to maintain. Of course, there are exceptions in which facilities opt to remove the plates and frames and clean them out of place in a washer similar to a centrifuge bowl. In such a case, the complexity (and cost) between the two technologies are more comparable; however, complex automation or cleaning out of place is not a common practice with filter presses.
Simpler technology: Manual filter press
Cycle time
The plasma fractionation process, like many pharmaceutical and industrial processes, requires substantial upfront investment by the manufacturer in the raw material. Facility throughput is a key consideration in achieving a cost-effective operation in a competitive market. The overall throughput of a facility relies on how quickly systems can be operated, cleaned, and used again.
The required time to operate a given system and then subsequently clean and prep that same system so it is ready for use again is collectively referred to as “cycle time”. Reasonable cycle times ensure the best use of a facility while maintaining a reliable and efficient schedule to maximize the return on investment. Before choosing a separation method, ask: ‘How long does each separation operation take? And how quickly can a facility turn it around for the next batch coming down the line?’
Cycle time is interrelated to operator interaction. The manual harvesting and cleaning of either method drive cycle time. Because manual operations are not as predictable due to the human factor, it is important to look at both the variability of and duration of the overall cycle time of each unit operation relative to maintaining a predictable throughput schedule.
While both methods require manual harvesting, the cleaning process differs substantially. Filter press plates can be sprayed down or cleaned in-place, while a centrifuge bowl needs to be manually disassembled, wheeled down to the washer, put in the washer, washed, taken out, brought back, and then re-assembled. Factor in the likely need for multiple centrifuges, and the cycle time becomes a significant factor.
Shorter cycle time: Filter press
Speeding up the process
How are some manufacturers finding workarounds to long cycle times? Buying doubles! There is a growing trend where manufacturers are buying an additional centrifuge bowl so they can use one bowl, break it apart, put it in the washer and have the second bowl ready to go. This effectively eliminates washing time from the critical path. However, a single centrifuge bowl is extremely expensive in the range of $500K, plus additional carts.
Similarly, some manufacturers who use a filter press method are buying spare plates and frames to cut out the times involved with cleaning. Others are installing two filter presses side-by-side that are tied into the same process system. They operate one and then switch to the other one while the first is harvested and cleaned, which drastically improves overall cycle time.
Where do we go from here?
As discussed, some of the current methods used in plasma fractionation date back to the 1940s. Undoubtedly, these classic methods will be updated or replaced as new technology comes along.
There are many benefits to be seen with the future of filter press automation as well. Automated filter presses that automatically harvest proteins and clean plates could drastically alter processing speed and be a game changer for the plasma fractionation industry. These are common in non-pharma processes (such as wastewater treatment plants), and some are now being designed with pharmaceutical applications in mind. Once manufacturers start to produce automated filter presses that are suitable for clean room installation, it is possible that filter presses could supersede centrifuges across all categories.
There are many other proven alternative centrifuge and filtration designs that have been around for years and have strong footholds in other pharmaceutical processes, but still see limited application in plasma fractionation. These include, but are not limited to: inverting basket and peeler centrifuges, agitated nutsche filters, and self-discharging multi-leaf filters. Process development around these already established technologies used in other similar applications could provide an easier path to change in the plasma fractionation industry.
Until then, manufacturers can look at capital costs, facility footprint, complexity, and cycle time to choose between the separation systems in common use in the plasma fractionation industry.